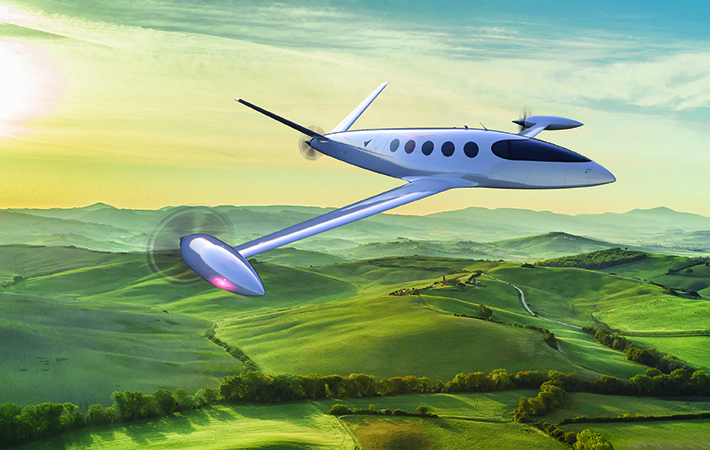
Green Skies Ahead
Years ago, an advertising campaign urged travelers to “Fly the friendly skies.” The efforts of a dedicated group of aviation industry stakeholders and forward-thinking ASTM International committee members may someday add the word “sustainable” to that phrase.
But what does the concept of sustainability even mean in the context of air travel? It reflects the fact that from an environmental standpoint, the skies are not especially friendly these days.
For example, the International Air Transport Association (IATA) estimates jet fuel consumed by commercial air travel accounts for about 2% of global carbon emissions. Other byproducts of commercial flight — contrails, for example, which are believed to affect cloud formation that contributes to atmospheric warming — push that percentage closer to five. And this share of total emissions is expected to increase further as more and more gas-powered vehicles are replaced by electric cars and trucks — thereby decreasing the share for automobiles.
IATA has also calculated that aviation sector CO2 emissions grew from 733 million to 915 million metric tons between 2013 and 2019. Another industry group, the International Civil Aviation Organization, anticipates that global international aviation emissions this year will be 70% greater than 15 years ago, and will be 700% greater by 2050.
READ MORE: The Promise of Urban Air Mobility
As with ground-based transportation, shifting to a different power source could be one way to mitigate these negative environmental effects. And even though the evolution of electric-powered aircraft is still in its infancy — and a time when large passenger jets exchange fuel lines for extension cords is still far down the road — many companies around the world are exploring the potential of alternative propulsion systems for smaller planes.
The aviation-related committees of ASTM International are right in the middle of this activity. The committees are working in two specific, and vitally important, areas: incorporating electric propulsion units (EPUs) into aircraft designs, and boosting the likelihood that passengers and first responders survive an electric-powered airplane crash.
A Long History in Aviation
ASTM International has been a valuable partner to aviation professionals for decades. The committee on aerospace and aircraft (F07) formed in 1969 and was the first committee to focus specifically on the industry. Since then, they have been joined by the committees on light sport aircraft (F37), unmanned aircraft systems (F38), aircraft systems (F39), and general aviation aircraft (F44).
Together, these committees are responsible for dozens of standards covering everything from F37’s specifications for design and performance of a light sport airplane (F2245), and F44’s specification for aircraft handling characteristics (F3173), to F39’s standard practice for inspection (F2696) and maintenance (F2799) of aircraft electrical wiring systems, and much more.
Not surprisingly, however, most of these standards apply to conventional airplanes that rely on internal combustion engines, either reciprocating or turbine, which burn fuel to provide propulsive power. Only in recent years has technology advanced to the point where electric motors can propel an aircraft large enough to carry passengers.
“What we’ve seen in the last decade is two trends: Smaller aircraft — unmanned aerial vehicles — that are powered by electric motors, and a decrease in the cost and size of the electronics required to drive electric motors,” says Herb Schlickenmaier, an industry consultant and member of both F44 and F39. “Those two trends have started to intersect in a general way, and it makes electric motors that can power a small aircraft and carry people possible now.”
Recognizing the viability of electric power systems for aircraft, the subcommittee on design, alteration, and certification of electric propulsion systems (F39.05) developed and gained approval in 2018 for the specification for design of electric propulsion units for general aviation aircraft (F3338). This foundational standard is now in the process of being updated.
Distributed Electric Propulsion One of the most promising advances in sustainable aviation technology is distributed electric propulsion (DEP, sometimes also referred to as DPS, or distributed propulsion systems). This innovative approach uses the aforementioned EPUs, which are small motors that enable aircraft to take off and land in small areas. They generate thrust distributed across the wing to modify the aerodynamic characteristics of an aircraft’s lifting surface and can enable improved cruise performance in planes with high-aspect-ratio wing configurations, such as NASA’s model X-57 airplane discussed later. With a different design, DEP motors can also enable lower take-off and approach speeds, allowing an aircraft to operate on smaller runways.
EPUs comprise an inverter (which converts DC to AC), a motor controller, and an electric motor that converts electrical energy (typically from a battery) into propulsive power. Unlike typical gas engines, they can be placed, sized, and operated with greater flexibility to provide improved performance.
“Electric propulsion provides a small, sufficiently powerful motor that can be placed in locations on the vehicle that would be difficult to do with internal combustion engines or turbine engines. Fuel lines are more difficult to route than electric wiring,” Schlickenmaier says.
William Fletcher is an airworthiness engineer with Rolls Royce, a leading jet engine manufacturer. He’s active in the F39.05 subcommittee that is working on revisions to the F3338 specification for general aviation aircraft to reflect the latest EPU configurations. “When the standard was first published, in looking at the industry needs, all of the electric motors and EPUs at the time were air-cooled,” he notes. “So in order to make the standard practical to get out and use immediately, the committee elected to leave the more complex technology out.”
Now, however, as Fletcher points out, a second wave of EPU designs feature higher power density, which requires a different method of cooling. (Power density refers to the amount of electro-mechanical power generated per unit mass of the motor.) “It’s hard to get high power density with an air-cooled motor, so this next wave of motors is going to use liquid cooling instead of air cooling,” he says.
There are already other ASTM International standards that address aircraft cooling and lubrication systems that the subcommittee can build on. Many were developed before the shift to performance-based regulations that began with passage of the U.S. National Technology Transfer and Advancement Act in 1996. This law represented a move away from a more prescriptive, government-directed approach to one that relies on industry stakeholders and standards development organizations like ASTM International to work through issues and achieve consensus.
“With liquid-cooling requirements, we’re capturing how the regulators expect the system to be designed and what features it should have,” Fletcher says. “So if it’s an oil-cooling system — particularly if it also cools the motor and the bearings — you still want to have oil filters to capture any metallic debris that bearings generate. With the new performance-based regulations being considered by the FAA and EASA, they leave how we’re going to meet those requirements up to organizations like ASTM.”
Another evolving element of the EPU standard relates to thrusters. Currently, F3338 only covers the electrical motor and does not include mechanical aspects (such as a propeller) to convert the motor’s output to thrust for propelling the aircraft. “They provide an interface where the motor output shaft can be connected to a separately provided thruster,” says Chris Eick, a member of F39.05. To be of practical use, the ASTM standards need to include the integration of propellers (fixed or variable pitch) or fans with the electrical motor currently defined by the EPU standard. This gap is currently being addressed by work items to revise F3888.
However, some EPUs are being designed with an integrated motor and propeller assembly (fixed or variable pitch) that cannot be separated. One of the current work items in the subcommittee is a revision to F3338 to address such next-generation EPU designs.
FOR YOU: Parachute Standard Supports a Safe Landing
Fletcher says that other parts of the F3338 standard focus on performance. For example, an endurance test requires that a motor be tested under realistic conditions for a certain number of hours to show that it functions properly. “Those requirements already exist in the standard, so even though we’re adding another system, such as liquid cooling or thrusters, the testing leg is satisfied by what’s in the existing body,” he says.
The X Factor
Specific electric-motor attributes and configurations are of course central to any discussion of DEP standards. But what about the performance of actual airplanes that use such systems? NASA’s first all-electric experimental plane, the X-57, is helping point the way to some answers.
The X-57 features a conventional fixed-wing design that incorporates 12 small, electrically driven propellers mounted on the leading edge of the wing and two large electric propellers at the wing tips. “We very much intend for the X-57 project to help drive the development of new standards with respect to distributed electric propulsion technology,” says Nicholas Borer, advanced concepts group lead in NASA’s Aeronautics Systems Analysis Branch, and a member of F39 and F44.
To that end, “NASA is studying the potential benefits of a distributed electric propulsion system in flight,” explains Borer’s colleague Sean Clarke, principal investigator for the X-57 project and a participant in the F39 and F44 committees. “As we build the technologies into our vehicle and conduct flight-performance testing, we are learning lessons and overcoming technical challenges that other designers of aircraft featuring electric propulsion technology will also face. ASTM standards are a key beneficiary of this information as we can work in the consensus-based environment to ensure the data from NASA is relevant and actionable.”
“The goal of our broad participation is to facilitate the critically needed technology transfer that will support the development of electric and distributed electric propulsion,” says Vince Schultz, X-57 deputy project manager and another member of both F39 and F44. “We are also sharing the data and best practices that can contribute to a common understanding of this new technology, which can be used by the FAA [U.S. Federal Aviation Administration] and other regulators worldwide in support of their certification efforts.”
In addition to F3338, revisions of two other EPU standards from the general aviation aircraft committee are underway. In fact, the specification for aircraft electric propulsion systems (F3239) is already on its second revision. “The revision includes system design principles for electric propulsion architectures that advise designers on how to ensure redundancy of critical features, best practices for integration of these technologies on aircraft, and hazard mitigation techniques,” Clarke says.
The specification for electrical systems for aircraft with electric or hybrid-electric propulsion (F3316) was approved by the general aviation aircraft committee in 2018 and provides guidance on how to ensure a smooth interface between a plane’s avionics systems and EPUs. Clarke points out that a vital element of this standard concerns monitoring and control of energy storage systems, such as the batteries for propulsion.
Protecting Passengers and First Responders
Uninterrupted battery performance is central to maintaining an electric aircraft’s power supply, and their weight and location within that aircraft — as well as the basic fact of their presence — must be accounted for if it should crash.
READ MORE: Back to the Moon
The subcommittee on design, alteration, and certification of electric propulsion systems (F39.05) is addressing these issues with ongoing collaboration among public interest organizations like NASA, civil aviation authorities including FAA and EASA (European Aviation Safety Agency), and producers eager to use these technologies. The focus is on a proposed specification for design of electric propulsion energy storage systems for general aviation aircraft (WK56255).
NASA’s Clarke notes the technical challenges of safe battery storage. “Current certification authority guidance requires batteries that are designed to contain complete failure of every cell in each battery compartment, but this has not been practical for large batteries, which are required for primary propulsion,” he says. “The X-57 uses a battery system based on design techniques NASA developed for the International Space Station, but on a much larger scale, and is intended to limit individual cell failures and prevent them from propagating to the rest of the compartment.”
Maintaining integrity within the battery pack is crucial to keeping an electric plane in the air. But what if the unthinkable happens, and there is a catastrophic failure in flight?
“Electric aircraft crash too, because they are still operated by pilots, and at the moment, these are small airplanes that are mostly flown by people who don’t fly every day,” says Tine Tomažič, director of research and development for Pipistrel, an aircraft manufacturer based in Slovenia that first started working with electric propulsion in electric-powered flight trainers like the Alpha Electro.
Once they began looking at post-impact conditions, Tomažič and his colleagues on the powerplant subcommittee (F44.40) created a checklist of sorts, with one area of particular scrutiny being the sheer weight of the battery pack. Steps must be taken to ensure that this extremely heavy assembly doesn’t shift and breach the passenger compartment during a crash, which could injure occupants.
Another critical item on an aircraft’s checklist is signage. Tomažič emphasizes its importance when that plane features a non-traditional power system. “First responders may have a hard time recognizing an electric airplane,” he says. “Maybe there’s no exhaust pipe, but that’s pretty much it. It’s difficult to tell.”
So the question becomes: How do you label an electric airplane so that first responders know where unique components are located and they understand the potential danger? “Sometimes it’s little things, like orange stickers on the outside of the aircraft that show where the cables are, so you don’t cut through them,” Tomažič says. “The notion is really not to discuss only the pilot and the occupants of the airplane. We also have to inform people around the airplane, at the airport, even potential bystanders who may help out.”
From signage guidelines to specifications for existing technology used in new ways, ASTM International standards will play a critical role in the push toward a more sustainable aviation ecosystem. Clarke puts it this way: “ASTM subcommittees have been a key forum for developers, certification authorities, and researchers to establish a common approach to making these technologies available to the public, leading to future aircraft that may be less expensive to own and operate, have lower environmental impact, and are more reliable.” ■
Jack Maxwell is a freelance writer based in Westmont, New Jersey.
U.N. Sustainable Development Goals Supported: