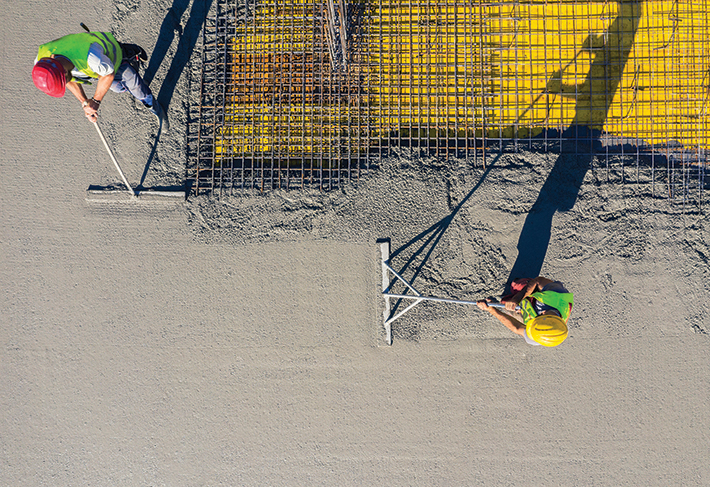
New Cementitious Materials Aid in the Quest for Net-Zero
“Embodied energy” sounds like one of those free-floating blobs you sometimes see in older science-fiction movies. But it’s actually a straightforward concept that attempts to quantify the total amount of energy it takes to produce an object or material, serving as a key metric for assessing the environmental impacts of the production process.
Take cement, for example. It’s the single most irreplaceable ingredient in concrete, reacting with water (and other ingredients) to create a versatile, durable construction material. The energy used to manufacture cement represents by far the largest single contributor to the overall embodied energy of concrete.
Given the fact that concrete is generally considered the second most widely used material on earth after water, and that cement and concrete are inextricably linked, the impact of reducing cement’s overall embodied energy on broader efforts to curb greenhouse gas emissions becomes very clear.
ASTM International is a key contributor to this work, and the committees on cement (C01) and concrete and concrete aggregates (C09) have developed a number of important test methods, specifications, and other standards that support industry efforts to reduce the energy intensity of the cement production process.
READ MORE: New Concrete, New Standards
Existing standards as well as new ones currently in development will be critical, as will international initiatives based on these standards.
Embodied Energy
Consider the concrete footings of a typical residential dwelling. The embodied (also sometimes referred to as “embedded”) energy of a home’s essential support structure is the sum of the energy consumed during completion of the many steps that lead from raw materials to a finished foundation.
Major inputs include the energy required to extract limestone from the earth; fire the limestone in a kiln (along with other ingredients) to create clinker; pulverize the clinker to form cement; and ultimately, combine that cement with water and aggregates like sand or gravel to produce concrete. The unfinished concrete then must be transported by truck to the job site for installation, consuming diesel fuel along the way.
Clearly, the journey from raw material to final product requires a lot of energy, which in turn contributes to higher levels of atmospheric carbon dioxide (CO²). To address the issue, the Global Cement and Concrete Association (GCCA) published “Concrete Future: The GCCA 2050 Cement and Concrete Industry Roadmap for Net Zero Concrete” in 2021.
This document represents a commitment from 40 of the world’s leading cement and concrete producers to work toward the goal of balancing the amount of greenhouse gases emitted during the production process with the amount they remove. Actions intended to achieve this balance include:
- reducing the use of fossil fuels and increasing the use of alternative fuels
- more efficient concrete production
- improved efficiency in the design of concrete projects and the use of concrete during construction
- investment in technology and innovation
- increased clinker substitution
The last action item referencing clinker is particularly important, and is one of the focal points of ASTM committee activity. The reason is that the vast majority of carbon dioxide emissions associated with concrete result from the production of clinker.
The Importance of Clinker
Clinker is the precursor to cement. It is produced in a rotating cement kiln, where ground limestone and other materials (like shale or clay) are heated to form pellets. The clinker pellets are then ground to a fine powder along with gypsum to create a mixture known as portland cement, the material that reacts with water to bind the various components of concrete together.
“Limestone is calcium carbonate, and the tremendous heat of the kiln breaks it down to its chemical components, calcium oxide and carbon dioxide,” explains Larry Sutter, Ph.D., vice chair of both C01 and C09, an active member of a number of related committees and subcommittees, and emeritus professor at Michigan Technological University.
New cementitious materials will bring industry closer to achieving net-zero carbon emissions.
A lot of energy is required to achieve the high temperatures necessary for these physical and chemical reactions to take place – up to 1450 Celsius – and maintain them continuously while the process unfolds. Important steps are being taken to address this major contributor to the total embodied energy of finished concrete.
“The cement industry has made significant reductions in CO² generated by burning fossil fuels,” Sutter says. “Alternative fuels are being used and electric kilns are being developed. However, the ability to switch to alternative fuels is limited, and new kiln technology such as electrification is still in its early stages.”
These nascent technologies show promise, but they only address one source of CO² emissions. Most of the CO² generated by the clinker production process is actually a byproduct of the chemical/physical process by which calcium carbonate (CaCO3) is converted into calcium oxide (CaO), which releases carbon dioxide into the atmosphere.
The confounding issue is that these CO² emissions are inevitable when limestone is used as the source of calcium – and limestone is the most plentiful source of calcium on Earth. Sutter acknowledges new cement production technologies based on calcium sources other than limestone, but adds that they have a long way to go before they can be scaled up.
The good news is that ongoing work on alternative, less energy-intensive forms of cement is yielding exciting results, bolstered by standards that help pave the way to wider usage of these new options.
Hydraulic or Nonhydraulic?
Non-hydraulic cement is a promising alternative to the hydraulic cement most commonly used around the world today.
“Hydraulic cements harden when a chemical reaction occurs between water and the anhydrous cement powder, forming water-insoluble hydrates that bind aggregates together into concrete,” says Rich Szecsy, Ph.D., C09 chair and executive vice president at Alamo Concrete. “In contrast, non-hydraulic cements harden through chemical reactions that do not include water as a reactant. One such reaction is carbonation.”
What does “carbonation” mean in this context? A small amount of water mixed with CO² gas turns into a mild carbonic acid that causes the release of calcite, which binds with CO² and immediately transforms it into solid calcium carbonate. So not only do non-hydraulic cements require about 80 percent less water to harden, they also actually capture CO² during the manufacturing process.
“Simplistically stated, the reaction is the opposite of the limestone reaction, meaning a source of calcium oxide or calcium silicate reacts with carbon dioxide to form calcium carbonate,” says Sutter. “So in addition to avoiding much of the CO² from the clinkering process, the hardened concrete also sequesters CO² through the hardening process.”
Sutter points out that non-hydraulic cements are significant because they either do not use the clinkering process, thereby avoiding CO² emissions, or if they do use the clinkering process, it is substantially different and emissions are greatly reduced.
Cement Alternatives
As cement continues to evolve toward a less energy-intensive future, ASTM standards are keeping pace.
Take the standard specification for portland cement (C150). According to Szecsy, prior to 2020, the dominant cement for general concrete construction in the U.S. conformed to this standard. Now, however, Type IL cements that meet the standard specification for blended hydraulic cements (C595) are the most commonly used. “The primary difference is that Type IL contains up to 15 percent limestone fines as part of the cement, a formula that helps reduce emissions during cement production,” he says.
Even as legacy standards are supplanted by newer ones that reflect incremental improvements in existing technology, development of specifications for alternatives like non-hydraulic cements continues to move forward. “Committee C01 recently added a standard specification for cements that require carbonation curing [C1905],” notes Szecsy. “These types of cement are produced by alternative technologies that do not eliminate CO² emissions, but significantly reduce them.”
Another path to lower energy intensity involves actually replacing a portion of the clinker used to manufacture concrete with alternatives known as supplementary cementitious materials (SCMs). Cesar Constantino emphasizes that in addition to reducing the total clinker content needed in concrete, SCMs can improve the concrete’s performance.
“This means that concrete can have a reduced carbon footprint when compared to mixes that are batched with 100 percent portland cement,” says Constantino, Ph.D., former chair of the ASTM Board of Directors, active member of a number of committees, and Resilience Action Fund Latin America ambassador. “At the same time, it can exhibit equal or better workability, pumpability, finishability, and flowability; lower bleeding and higher strengths; and improved resistance to sulfates, alkali-silica reaction, and steel-reinforcement corrosion, among other benefits.”
SCMs are not a new idea. Industrial waste products like fly ash, slag cement, and silica fume have been utilized in this way for decades, Szecsy says, with support from standard specifications for slag cement for use in concrete and mortars (C989); silica fume used in cementitious mixtures (C1240); and coal fly ash or raw or calcined natural pozzolan for use in concrete (C618). Pozzolans comprise a broad class of SCMs that, when mixed with other sources of calcium, react chemically to form compounds with cementitous properties.
Fly ash is currently the most common type of SCM. But the ongoing decommissioning of coal-fired power plants — one important source of this material — has led to availability issues in some areas. “C09 is working on a performance-based specification that will allow for the use of new SCMs that are poised to fill these gaps,” Sutter says.
Several companies are exploring the potential of different types of SCMs that they say lower energy intensity and/or the overall CO² burden brought into the concrete.
“Firms are looking at different ways to produce cements that replace high-energy-requirement processes, or ways in which CO² from the atmosphere can be used during the curing process of the concrete mixes to serve as CO² sinks and lower the net emissions attributable to the cement,” says Constantino.
Performance-Based vs. Prescriptive
When it comes to standards, most stakeholders prefer performance-based specifications to prescriptive specifications, which place a given material into a defined category based on chemical or physical properties. In the case of concrete, a prescriptive specification would prescribe a mixture – for example, minimum cement content or limits on total percentage of SCMs – with little room for variation. Performance is implied based on those properties.
By contrast, performance-based specifications present clear, measurable, and enforceable functional requirements for materials. The absence of chemical and compositional limits allows different materials to be specified as long as they offer the same performance.
FOR YOU: 5 Standards That Support Resilience
“Performance specifications provide opportunities for innovation,” Sutter says. “Rather than prescribing a specific material for use in concrete, a performance specification states what the attributes of the final product need to be and allows the producer the opportunity to use innovative technologies to achieve the specified performance.”
The standard performance specification for blended hydraulic cements is one of more than 100 ASTM standards related to the ubiquitous building material. But to Constantino, it’s one of the most important, both in the U.S. and internationally.
“This specification offers flexibility in lowering clinker usage from 90-plus percent when compared to portland cement,” he says. Constantino adds that the adoption and use of C595 outside the U.S. has already proven valuable in lowering the net CO² burden of finished cements by, for example, enabling the use of natural pozzolans to reduce clinker production.
Latin America
Matías Polzinetti echoes Constantino’s comment regarding the international impact of standards like C595 and the standard performance specification for hydraulic cement (C1157). The designated liaison between ASTM and the Colombia-based Inter-American Concrete Federation (FICEM) notes that his organization signed an agreement with ASTM in May 2022 to establish a framework for cooperation in efforts to achieve technical standardization in cement and concrete.
“As FICEM works to lead the cement industry in Latin America and the Caribbean toward carbon neutrality by 2050, the right regulatory frameworks for decarbonization, as well as strategic alliances, are elements of central importance,” Polzinetti says. “In particular, we believe that our alliance with ASTM can feed back into the work of committees C01 and C09.”
In Polzinetti’s experience, ASTM standards are the main reference for the development of local standards. “Among the 17 countries surveyed in the region, only four use a precedent other than ASTM. In the case of countries that have adopted standards with a performance approach, such as Guatemala and Colombia, they have done so in the same way as C1157.”
Manuel Lascarro, executive secretary of the Ibero-American Federation of Ready Mixed Concrete (FIHP), has also seen ASTM’s impact. “The Colombian Institute of Technical Standards and Certification (ICONTEC) has a Memorandum of Understanding with ASTM to facilitate access to documents and experts in the field,” he explains. It’s also worth noting that ICONTEC’s executive manager, Roberto Montoya, just began a two-year term on the ASTM Board of Directors.
The Colombian concrete construction code (NSR-10) and the local standard for cement (NTC-121) are based on C150, C595, and C1157. “NTC-121 is a standard oriented to reach specifications by performance, and has helped to reduce the carbon footprint of cement by an average of 27 percent compared to ordinary portland cement,” says Lascarro.
Constantino ends on a hopeful note. “As an international standards-development organization, ASTM is in a unique position to serve as the convening forum where global wants and needs in the decarbonization space for cement and concrete can be addressed, and where an open, transparent, consensus-based process can help expedite the adoption of emerging technologies through a research-to-standards approach.” ■
Jack Maxwell is a freelance writer based in Westmont, N.J.